Cutting tool materials have seen only a few significant advancements in the past 100 years or so, such as the introduction of high-speed steel in the 1800s and cemented carbide in the middle of the 20th century. Today, cemented carbide still dominates the market for basic turning and milling while HSS continues to rule the tap and drill markets.
It is difficult to identify other products or technologies of that age that remain applicable in modern manufacturing. Technology that old normally has been obsolete for decades. Contemporary machining has seen the introduction of advanced materials like ceramic, polycrystalline diamond and cubic boron nitride, but these materials do not have the same broad range of applications as cemented carbide and HSS.
So how does a century-old technology remain viable at present-day machine shops? There are a few reasons. Cutting tool manufacturers have altered chemistry to improve performance and mitigate weaknesses. In other cases, manufacturers have employed advanced grinding techniques for solid tools and have improved pressed geometry for inserts. Both approaches are effective. And when combined, they offer countless combinations for creating cutting tools. These combinations routinely are amended and presented to the market with catchy names as the latest and greatest cutting tool advancements.
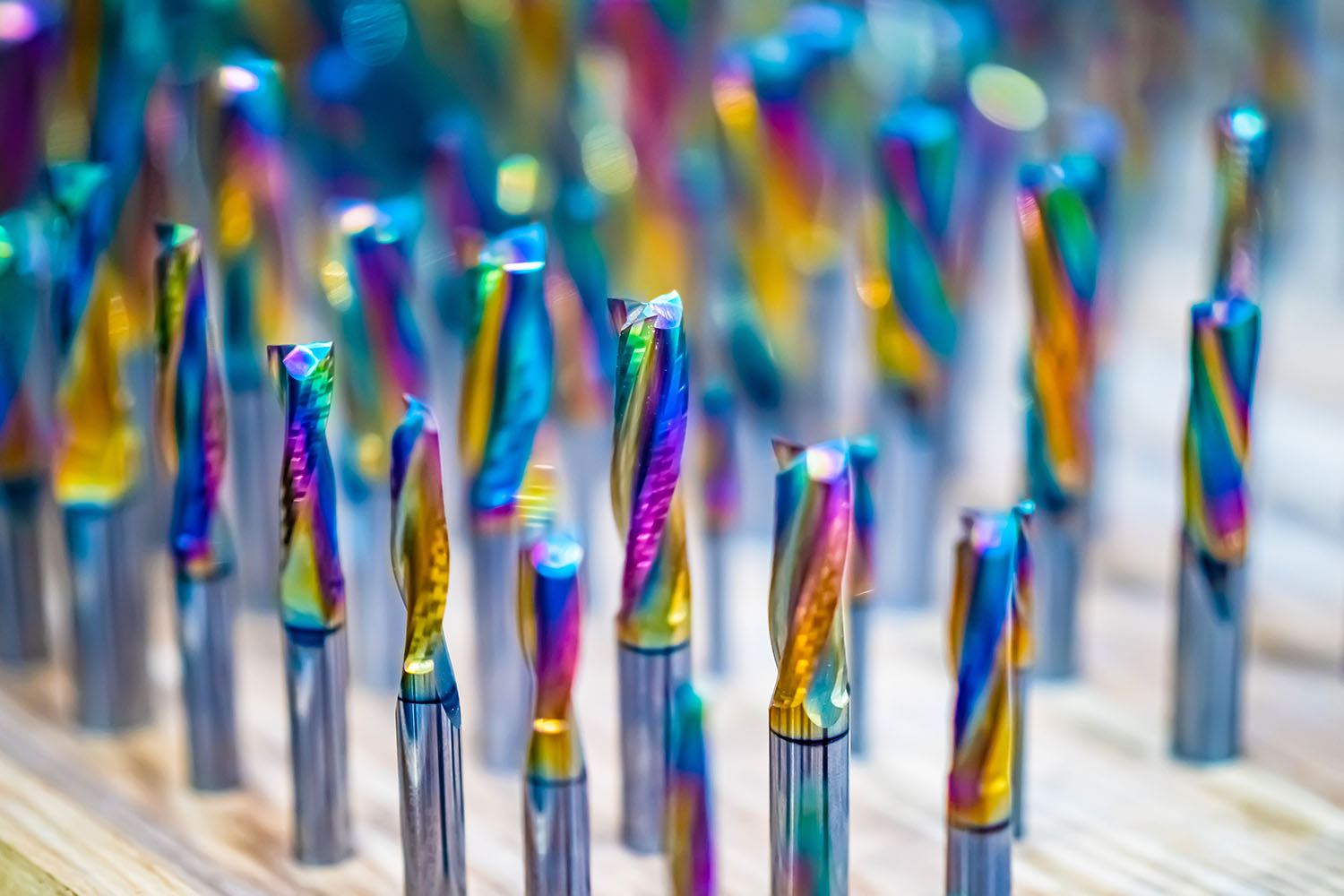
The third and probably most effective advancement has been the development of coatings that are applied to cutting tools. Working to keep up with demand for improved productivity and performance, cutting tool manufacturers started applying coatings to tools in the 1960s. Application of coatings was a step change in cutting tool technology, much like the shift from HSS to cemented carbide.
Coatings are applied using one of two processes: chemical vapor deposition or physical vapor deposition.
CVD processes are performed under very high temperatures, and the coating materials react chemically with the material of the cutting tool. Because they are bonded through chemical reactions, CVD coatings have strong adhesion to the tool. Thus, they can be applied in thick layers. CVD coatings tend to be stronger, and the thicker layers provide an excellent heat barrier to the cutting edge.
PVD coatings are applied at lower temperatures than CVD and do not bond through chemical reactions. Rather, PVD coatings are deposited on the surface of the tool, forming a protective layer. Lower application temperatures preserve the mechanical properties of sensitive cutting tool materials. PVD coatings are applied in thinner layers and, unlike CVD, do not reduce the keenness of the cutting edge.
The application of coatings greatly enhances the qualities of the cutting tool by improving lubricity, increasing hardness and insulating the tool from elevated temperatures. These improvements ultimately translate into increased tool life and higher removal rates.
Like putting oil on moving parts, the increased lubricity from the coating reduces friction between the chip and the rake face of the tool. By reducing friction, heat produced during chip formation is reduced, which increases tool life. Lower temperatures in the shear zone reduce chemical reactions that would result in wear mechanisms like cratering. Lubricity also fights built-up edge, in which the material being machined adheres to the cutting edge. BUE increases heat in the shear zone and is the primary cause of failures, such as edge fractures.
Coating materials are much harder than tool materials. Higher hardness means that the tool can operate at higher speeds than the same uncoated tool. Like improved lubricity, increased hardness fights abrasive wear that would result in cratering. Without coatings, it would be very difficult to machine green ceramics, carbon fiber and similar materials used in advanced applications.
Every machinist knows, usually from bad experiences, that heat kills tools. Coatings improve wear resistance and reduce heat by providing an insulating barrier to the surface of the tool, helping fend off heat-caused failures like plastic deformation. This attribute is especially important when using HSS tools, which are already more susceptible to heat-induced failures than carbide is.
Coatings improve productivity and reduce tool costs, but coatings are application-specific. Using a coating that is not designed for a workpiece material can cause the same failure modes that coatings are intended to combat.
So which coatings should you use? Honestly, you do not need to worry about it.
Your favorite turning and milling insert grades already are coated, often with multiple layers to take advantage of properties from different coatings. I have seen uncoated carbide inserts but not in many years. If those inserts still exist, they are available deep inside catalogs and so few in number that we fairly can say all carbide inserts are coated.
Unlike inserts, uncoated solid tools like endmills and drills are common, so there is decision making to be done when buying these tools with coatings. But again, do not worry. Manufacturers have teams of materials engineers who determine application criteria. A search of your favorite manufacturer’s website or a phone call to the application folks will put you on the right track.
The moral of this story is simple: Coated tools improve machining processes by combating multiple failure modes. However, coatings are application-specific and must be paired with workpiece materials to realize the benefits.
Related Glossary Terms
- abrasive
abrasive
Substance used for grinding, honing, lapping, superfinishing and polishing. Examples include garnet, emery, corundum, silicon carbide, cubic boron nitride and diamond in various grit sizes.
- built-up edge ( BUE)
built-up edge ( BUE)
1. Permanently damaging a metal by heating to cause either incipient melting or intergranular oxidation. 2. In grinding, getting the workpiece hot enough to cause discoloration or to change the microstructure by tempering or hardening.
- built-up edge ( BUE)2
built-up edge ( BUE)
1. Permanently damaging a metal by heating to cause either incipient melting or intergranular oxidation. 2. In grinding, getting the workpiece hot enough to cause discoloration or to change the microstructure by tempering or hardening.
- ceramics
ceramics
Cutting tool materials based on aluminum oxide and silicon nitride. Ceramic tools can withstand higher cutting speeds than cemented carbide tools when machining hardened steels, cast irons and high-temperature alloys.
- chemical vapor deposition ( CVD)
chemical vapor deposition ( CVD)
High-temperature (1,000° C or higher), atmosphere-controlled process in which a chemical reaction is induced for the purpose of depositing a coating 2µm to 12µm thick on a tool’s surface. See coated tools; PVD, physical vapor deposition.
- chemical vapor deposition ( CVD)2
chemical vapor deposition ( CVD)
High-temperature (1,000° C or higher), atmosphere-controlled process in which a chemical reaction is induced for the purpose of depositing a coating 2µm to 12µm thick on a tool’s surface. See coated tools; PVD, physical vapor deposition.
- coated tools
coated tools
Carbide and high-speed-steel tools coated with thin layers of aluminum oxide, titanium carbide, titanium nitride, hafnium nitride or other compounds. Coating improves a tool’s resistance to wear, allows higher machining speeds and imparts better finishes. See CVD, chemical vapor deposition; PVD, physical vapor deposition.
- cratering
cratering
Depressions formed on the face of a cutting tool caused by heat, pressure and the motion of chips moving across the tool’s surface.
- cubic boron nitride ( CBN)
cubic boron nitride ( CBN)
Crystal manufactured from boron nitride under high pressure and temperature. Used to cut hard-to-machine ferrous and nickel-base materials up to 70 HRC. Second hardest material after diamond. See superabrasive tools.
- cutting tool materials
cutting tool materials
Cutting tool materials include cemented carbides, ceramics, cermets, polycrystalline diamond, polycrystalline cubic boron nitride, some grades of tool steels and high-speed steels. See HSS, high-speed steels; PCBN, polycrystalline cubic boron nitride; PCD, polycrystalline diamond.
- gang cutting ( milling)
gang cutting ( milling)
Machining with several cutters mounted on a single arbor, generally for simultaneous cutting.
- grinding
grinding
Machining operation in which material is removed from the workpiece by a powered abrasive wheel, stone, belt, paste, sheet, compound, slurry, etc. Takes various forms: surface grinding (creates flat and/or squared surfaces); cylindrical grinding (for external cylindrical and tapered shapes, fillets, undercuts, etc.); centerless grinding; chamfering; thread and form grinding; tool and cutter grinding; offhand grinding; lapping and polishing (grinding with extremely fine grits to create ultrasmooth surfaces); honing; and disc grinding.
- hardness
hardness
Hardness is a measure of the resistance of a material to surface indentation or abrasion. There is no absolute scale for hardness. In order to express hardness quantitatively, each type of test has its own scale, which defines hardness. Indentation hardness obtained through static methods is measured by Brinell, Rockwell, Vickers and Knoop tests. Hardness without indentation is measured by a dynamic method, known as the Scleroscope test.
- high-speed steels ( HSS)
high-speed steels ( HSS)
Available in two major types: tungsten high-speed steels (designated by letter T having tungsten as the principal alloying element) and molybdenum high-speed steels (designated by letter M having molybdenum as the principal alloying element). The type T high-speed steels containing cobalt have higher wear resistance and greater red (hot) hardness, withstanding cutting temperature up to 1,100º F (590º C). The type T steels are used to fabricate metalcutting tools (milling cutters, drills, reamers and taps), woodworking tools, various types of punches and dies, ball and roller bearings. The type M steels are used for cutting tools and various types of dies.
- lubricity
lubricity
Measure of the relative efficiency with which a cutting fluid or lubricant reduces friction between surfaces.
- mechanical properties
mechanical properties
Properties of a material that reveal its elastic and inelastic behavior when force is applied, thereby indicating its suitability for mechanical applications; for example, modulus of elasticity, tensile strength, elongation, hardness and fatigue limit.
- milling
milling
Machining operation in which metal or other material is removed by applying power to a rotating cutter. In vertical milling, the cutting tool is mounted vertically on the spindle. In horizontal milling, the cutting tool is mounted horizontally, either directly on the spindle or on an arbor. Horizontal milling is further broken down into conventional milling, where the cutter rotates opposite the direction of feed, or “up” into the workpiece; and climb milling, where the cutter rotates in the direction of feed, or “down” into the workpiece. Milling operations include plane or surface milling, endmilling, facemilling, angle milling, form milling and profiling.
- physical vapor deposition ( PVD)
physical vapor deposition ( PVD)
Tool-coating process performed at low temperature (500° C), compared to chemical vapor deposition (1,000° C). Employs electric field to generate necessary heat for depositing coating on a tool’s surface. See CVD, chemical vapor deposition.
- physical vapor deposition ( PVD)2
physical vapor deposition ( PVD)
Tool-coating process performed at low temperature (500° C), compared to chemical vapor deposition (1,000° C). Employs electric field to generate necessary heat for depositing coating on a tool’s surface. See CVD, chemical vapor deposition.
- plastic deformation
plastic deformation
Permanent (inelastic) distortion of metals under applied stresses that strain the material beyond its elastic limit.
- polycrystalline diamond ( PCD)
polycrystalline diamond ( PCD)
Cutting tool material consisting of natural or synthetic diamond crystals bonded together under high pressure at elevated temperatures. PCD is available as a tip brazed to a carbide insert carrier. Used for machining nonferrous alloys and nonmetallic materials at high cutting speeds.
- rake
rake
Angle of inclination between the face of the cutting tool and the workpiece. If the face of the tool lies in a plane through the axis of the workpiece, the tool is said to have a neutral, or zero, rake. If the inclination of the tool face makes the cutting edge more acute than when the rake angle is zero, the rake is positive. If the inclination of the tool face makes the cutting edge less acute or more blunt than when the rake angle is zero, the rake is negative.
- tap
tap
Cylindrical tool that cuts internal threads and has flutes to remove chips and carry tapping fluid to the point of cut. Normally used on a drill press or tapping machine but also may be operated manually. See tapping.
- turning
turning
Workpiece is held in a chuck, mounted on a face plate or secured between centers and rotated while a cutting tool, normally a single-point tool, is fed into it along its periphery or across its end or face. Takes the form of straight turning (cutting along the periphery of the workpiece); taper turning (creating a taper); step turning (turning different-size diameters on the same work); chamfering (beveling an edge or shoulder); facing (cutting on an end); turning threads (usually external but can be internal); roughing (high-volume metal removal); and finishing (final light cuts). Performed on lathes, turning centers, chucking machines, automatic screw machines and similar machines.
- wear resistance
wear resistance
Ability of the tool to withstand stresses that cause it to wear during cutting; an attribute linked to alloy composition, base material, thermal conditions, type of tooling and operation and other variables.