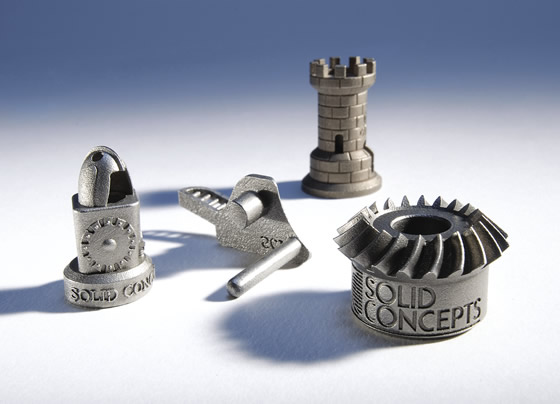
Courtesy of Solid Concepts
Sample parts made via direct metal laser sintering.
3D printing poised to turn prototyping on its head.
Engineers and product designers have more rapid prototyping choices than an aficionado at an art fair. Stereolithography (SLA), selective laser sintering (SLS), fused deposition modeling (FDM) and direct metal laser sintering (DMLS) are but some of the available additive manufacturing (AM) technologies, which cover a range of materials from nylon, polycarbonate, PEEK (polyether ether ketone) and polypropylene to aluminum, cobalt chrome and titanium.
Say you want to develop the latest and greatest garden gnome. For $600 or so you can log in to your Amazon account, order a 3D desktop printer and be creatingyard ornaments from ABS (acrylonitrile-butadiene-styrene) in no time. But if you’re planning to build a stainless steel army of those cute little dwarves, you’d better have some serious cash in the bank—a metal-capable machine easily costs $750,000.
If you’re not a do-it-yourselfer, there are plenty of service bureaus and specialty shops willing to build whatever design you have up your sleeve. Simply upload a CAD file to one of the many prototyping Web sites, enter credit card information and your latest brainchild will appear on your doorstep a few days later.
Goodbye Old School
It wasn’t always this way. Before 3D printers were developed, having a prototype made was a straightforward process—design the part, send the drawing out for a couple of quotes and award some lucky machine shop a purchase order. Depending on the part complexity, raw material lead time and the preferred vendor’s backlog, your shiny new prototype might arrive in a month or two.
A slow avalanche of change began in the mid-1980s when 3D Systems delivered the first SLA systems. The lure of shorter product development cycles was intriguing, but those early machines cost upwards of $350,000 and were able to manufacture parts from but a few flavors of photopolymer, thus limiting their functionality to foundry patterns and visualization for high-end product development.
As a result, SLA had little impact on machine shops, and traditional prototyping continued unabated for a few years more. Enter FDM, an AM technology that became increasingly prevalent during the early 1990s, one that offered a broader range of materials than SLA and a price tag within the reach of many capital equipment budgets. Still, machine shops had little cause to worry. After all, additive manufacturing was limited to plastic, and everyone knew that prototyping of functional metal parts would always require machining centers, lathes and EDM. Think again.
Big Changes Coming
Over the past few years, the metalworking industry has begun to experience a paradigm shift. DMLS and SLM have been under development even longer than plastic printing, but it wasn’t until recently that these metal-capable technologies became a viable alternative to machined prototypes. At the same time, the AM industry has seen increased consolidation of small prototype houses into “superbureaus,” with hundreds of machines nationwide. Never has the design community had so many choices for prototyping and low-volume production.
One of these is RedEye, a division of AM equipment manufacturer Stratasys Ltd. Product Manager Tim Thellin explained that the RedEye facility in Eden Prairie, Minn., has more than 80 FDM systems, with another 80 or so in operation worldwide. “There’s great interest in 3D printing,” he said. “We work with a number of industries, including automotive, aerospace, medical and defense. Together with our partner facilities, we can manufacture parts for most anyone, out of every thermoplastic material that FDM can produce.”
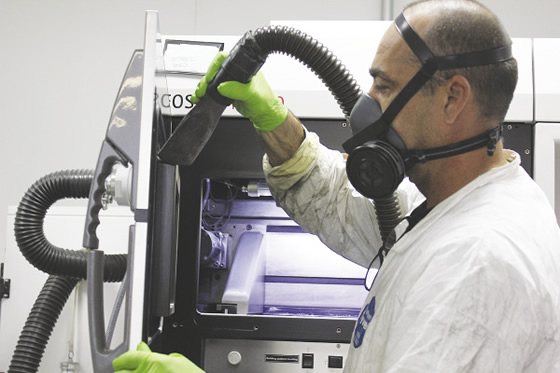
Courtesy of Linear Mold & Engineering
Linear Mold & Engineering performs maintenance work on an EOS DMLS machine.
Most of RedEye’s orders are still low volume, yet quantities have been on the rise as machines get larger and capacity has been added to support higher volume projects —production runs of 1,000 pieces or more are not unusual. According to Thellin, for anyone who needs engineering-grade plastic components with tolerances no tighter than ±0.005 " (±0.127mm), FDM is an excellent choice.
Thellin said it’s unlikely AM will be used to crank out millions of the same part, at least not in the foreseeable future. He maintains that AM should be considered a complement to traditional manufacturing processes, not a replacement. This is especially true with metal components. “DMLS is still nowhere near as accurate as machining and typically requires secondary finishing operations.”
Do Me a Solid
The DMLS arm of RedEye is Solid Concepts Inc. That’s not to say the company works exclusively with metal-based AM, however. Project Manager Chuck Alexander said the Valencia, Calif.-based firm is technology-agnostic, utilizing whatever is needed. This includes the full gamut of AM machines, as well as CNC machining and injection molding equipment.
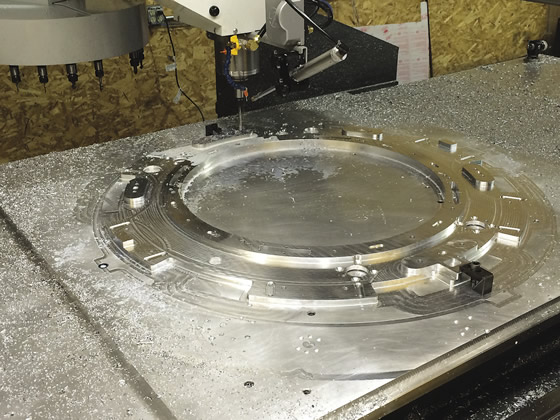
Courtesy of Pro CNC
Endmilling on a gantry-style machining center is one of the final manufacturing steps for this aluminum prototype.
Alexander warned that the manufacturing industry as a whole needs an education on AM’s capabilities. “The disruptive effect additive manufacturing has had on the market is based on how it changes the design process,” he said. “We’re just now starting with a generation of engineers somewhat familiar with additive and 3D printing and what it can do, but we still haven’t even scratched the surface of its potential. AM allows you to do things that you simply can’t do with traditional manufacturing methods.”
Nonetheless, there are some things traditional machining can do that additive manufacturing can’t, Alexander added. “For most applications, we see DMLS as a way to quickly achieve near-net shape, then use CNC machines to attain high-grade surface finishes, close-tolerance holes and precise feature locations. From an accuracy perspective, I compare AM to a precision casting process, where secondary operations are required for critical surfaces.”
Shopping Around
Someone who needs no education on AM’s capabilities is Steve Upton, president of Schmit Prototypes, Menomonie, Wis. Aside from the shop’s 18 CNC machines, there are also two SLA systems, an FDM and an Objet—a PolyJet 3D printer that operates much like a typical inkjet printer. Despite the shop’s large investment in AM equipment, much of the work done on those machines is internal, producing master patterns to create silicone molds for casting urethane parts. While Upton said that’s been the case for years, he noted that customer requests for rapidly built visual models (finished and painted or with only a light finish) have increased sharply. “Using new resins that mimic ABS or polypropylene, many parts that would have required machining before can now be built faster and with less cost via AM.”
Upton pointed out that the machine shop is quite busy, but rapid prototyping is becoming an increasingly competitive market, at least as far as AM-produced parts are concerned. “There are service bureaus with hundreds of machines. This tends to bring the profit margins down quite a bit, so we focus on more complex work and support the prototyping needs of customers with whom we have long-term relationships.”
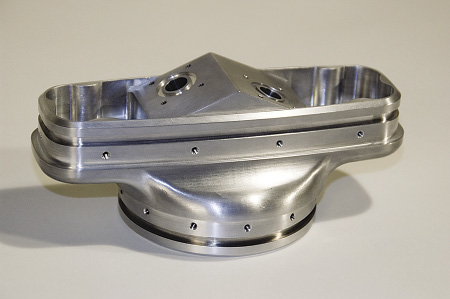
Courtesy of Pro CNC
Complex prototypes like this require multiple machining operations, fixtures and CAM programs.
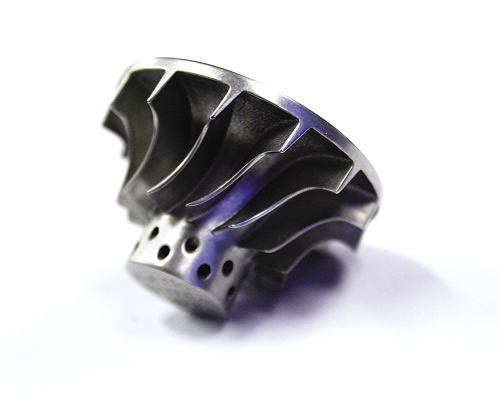
Courtesy of Solid Concepts
An impeller wheel is made via DMLS by Solid Concepts from cobalt chrome molybdenum.
Upton’s not alone. Hybrid job shops—those that have picked up a 3D printer or two in hopes of augmenting their existing CNC services with low-cost prototyping—may find it difficult to adjust to this new business model.
One of these hybrid shops is Pro CNC Inc., a contract assembly and vertically integrated CNC machine shop in Bellingham, Wash. Like Upton, Vice President Paul Van Metre said Pro CNC keeps its FDM machine busy servicing existing customers and building prototypes and fixtures for its own internal needs, but is not pursuing low-margin AM work.
“It’s become such a commoditized industry at this point that it doesn’t make financial sense to try and compete with a Web site where customers can load their CAD models and buy the resultant parts for barely more than the cost of the material,” Van Metre said. “This is certainly the case with many of the service providers, as their prices have really dropped over the last few years.”
This isn’t a case of sour grapes. All involved understand the math behind the operation of large numbers of mostly unattended machines—labor, burden and tooling expense can be amortized across more equipment, and with greater raw material volumes, costs go down. This is good news for consumers, and, even for Pro CNC, which occasionally uses another of Stratasys’ recent acquisitions, service bureau Harvest Technologies, for some high-volume work.
Old School for Medical
Despite AM’s increasing speed, accuracy and material offerings, there are some instances where it might never be used. Rick Paulson, general manager of Micro-Matics Inc., a Fridley, Minn., machine shop specializing in Swiss-style and conventional machining, said he has no 3D printers and probably never will. “We do offer a quick-response program for one-offs and low-volume work, but since most of our parts are for the medical and aerospace industries, we have to prototype them on the production machine. It’s a customer requirement.”
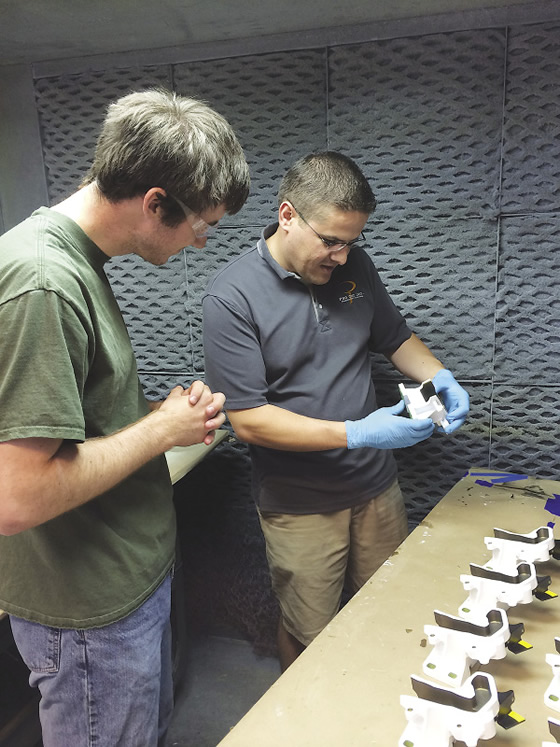
Courtesy of Pro CNC
Griffin Gamble (left), assembly technician, and Vince Ceausu, inspector, examine the paint job on a set of Boeing 787 airliner door fittings (below) cut on 5-axis machining center at Pro CNC.
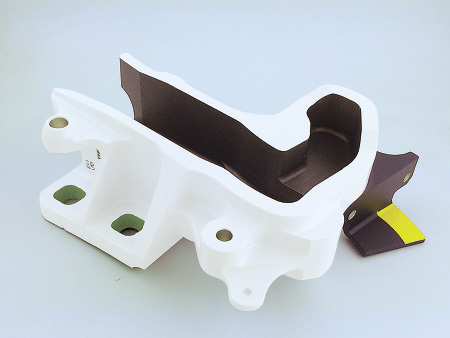
When the shop is asked to machine prototypes, typically on its Swiss-style machines, it produces quantities ranging from one to 50. Recently, one customer asked for prototype parts for a medical device that has been on the market for 4 years and is being redesigned. The order included three versions of five different parts.
“They want them all done on Swiss machines because that way, when they put the device together and it works, they’re going to move on to higher quantity prototypes, then validation parts and production parts. That’s pretty much the way those groups work. When the prototype is made on the production equipment, they know they won’t have any variation in their process.”
Even with this type of continuing business, Paulson sees the impact AM is having on shops around him. One of his suppliers, a cryogenic deburring house, makes fixtures on its 3D printer for holding parts during bead blasting. Another builds in-house inspection fixtures using AM, a huge cost savings compared to having them made at a tool-and-die shop. “There are big changes coming,” he said, laughing. “I even saw one of these things at Home Depot the other day. The technology is definitely going to get better, and the demand will increase.”
Consignment and Competency
Paul Parzuchowski would like to call his company the “Google” of additive manufacturing, providing information on AM to all comers. As the director of marketing and training for Linear Mold & Engineering Inc., Livonia, Mich., he’s been tasked with educating potential and existing customers on both the benefits and pitfalls of AM. “We’ve seen too many cases where a shop spends $750,000 or more on a machine and then takes months and months to figure out how to use it successfully,” he said.
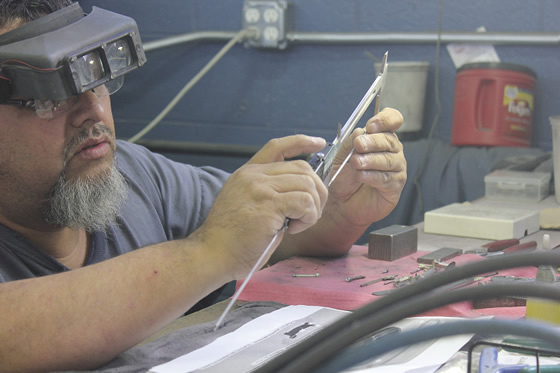
Courtesy of Linear Mold & Engineering
Measuring a laser-sintered workpiece at Linear Mold & Engineering.
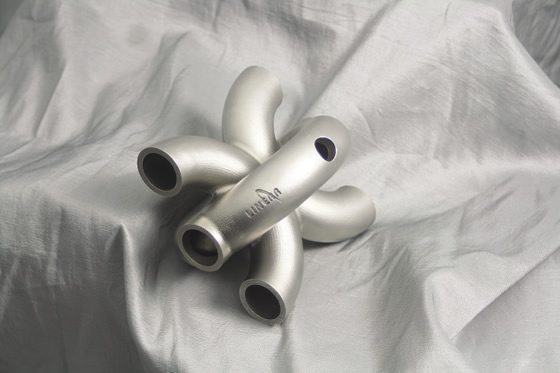
Courtesy of Linear Mold & Engineering
A test part with complex geometries made via DMLS.
To avoid this scenario, Linear offers technology transfer training—in 4½ days, would-be AM users learn how to build parts, from conception to completion. “Purchasing the machine is but a small part of the process,” Parzuchowski explained. “Of equal importance is understanding how to set up the build job to avoid failure, post-process parts and make the entire process cost-effective.”
This means learning the techniques for proper part orientation, running multiple jobs simultaneously and understanding build time and machine capacity. “If you want a snack, you don’t fire up the oven to bake one cheese stick,” he quipped. “You’ll load up an entire box, or else you’re wasting time and money.” How those cheese sticks are fixtured is also important—stand them up straight and you’ll achieve maximum use of oven space, but one wrong move and the entire batch could topple. Lay them flat and they might stick to the pan. It’s no different with AM.
Granted, it takes more than a week to become an AM master. If you’re not ready to make the capital investment, Linear will be happy to consign a machine to you, along with the people required to operate it. In either case, Parzuchowski said AM is expected to be a $20 billion global industry by 2020, with 10 percent of that in DMLS and SLM. “If you want to be considered a bona fide prototype shop, this is something you should at least be knowledgeable about, if not actively investing in. Ten years from now, I can’t imagine prototyping being done any other way.”
Exploding Market?
Charles Koch, owner of virtual manufacturing company 3Axis Development Inc., Boynton Beach, Fla., agreed. “3D metal printing is going to explode. If your shop makes a living on EDMing and 5-axis milling, you’ll be in trouble long-term as DMLS machines get bigger and more accurate.”
Koch, a sales representative for a number of rapid prototyping companies, said the industry is undergoing a monumental shift. Not only will this have a profound influence on manufacturing, but it will also change the way we live.
“The impact will be twofold,” he explained. “First, the small 3D plastic printer market is going to explode, and sub-$1,000 machines will work their way into all manner of businesses and even homes. At the same time, metal printing machines will drop in price—one that costs $1 million today will eventually be $100,000 or less. Both of these events will change the way people think about manufacturing.”
For example, Koch suggests in-home 3D printers will one day be used to manufacture all manner of household gadgets. Need a new picture frame or maybe a pipe wrench to tighten a leaky faucet? Just download the CAD files to your 3D printer and you’ll have the picture hung and water leak stopped before breakfast. No more driving to the hardware store or ordering parts online. “When we were living on the frontier, we had to make the things we needed to survive,” Koch said, “and 3D printing is the door to a completely new frontier. We’re just now at the beginning of it.” CTE
Contributors
Linear Mold & Engineering Inc.
(734) 422-6060
www.linearmold.com
Micro-Matics Inc.
(763) 780-2700
www.micro-matics.com
Pro CNC Inc.
(866) 477-6262
www.procnc.com
RedEye
(866) 882-6934
www.redeyeondemand.com
Schmit Prototypes
(715) 235-8474
www.schmitprototypes.com
Solid Concepts Inc.
(888) 311-1017
www.solidconcepts.com
3Axis Development Inc.
(561) 752-9095
www.3axis.us
Related Glossary Terms
- centers
centers
Cone-shaped pins that support a workpiece by one or two ends during machining. The centers fit into holes drilled in the workpiece ends. Centers that turn with the workpiece are called “live” centers; those that do not are called “dead” centers.
- chuck
chuck
Workholding device that affixes to a mill, lathe or drill-press spindle. It holds a tool or workpiece by one end, allowing it to be rotated. May also be fitted to the machine table to hold a workpiece. Two or more adjustable jaws actually hold the tool or part. May be actuated manually, pneumatically, hydraulically or electrically. See collet.
- computer numerical control ( CNC)
computer numerical control ( CNC)
Microprocessor-based controller dedicated to a machine tool that permits the creation or modification of parts. Programmed numerical control activates the machine’s servos and spindle drives and controls the various machining operations. See DNC, direct numerical control; NC, numerical control.
- computer-aided design ( CAD)
computer-aided design ( CAD)
Product-design functions performed with the help of computers and special software.
- computer-aided manufacturing ( CAM)
computer-aided manufacturing ( CAM)
Use of computers to control machining and manufacturing processes.
- electrical-discharge machining ( EDM)
electrical-discharge machining ( EDM)
Process that vaporizes conductive materials by controlled application of pulsed electrical current that flows between a workpiece and electrode (tool) in a dielectric fluid. Permits machining shapes to tight accuracies without the internal stresses conventional machining often generates. Useful in diemaking.
- endmilling
endmilling
Operation in which the cutter is mounted on the machine’s spindle rather than on an arbor. Commonly associated with facing operations on a milling machine.
- flat ( screw flat)
flat ( screw flat)
Flat surface machined into the shank of a cutting tool for enhanced holding of the tool.
- gang cutting ( milling)
gang cutting ( milling)
Machining with several cutters mounted on a single arbor, generally for simultaneous cutting.
- machining center
machining center
CNC machine tool capable of drilling, reaming, tapping, milling and boring. Normally comes with an automatic toolchanger. See automatic toolchanger.
- metalworking
metalworking
Any manufacturing process in which metal is processed or machined such that the workpiece is given a new shape. Broadly defined, the term includes processes such as design and layout, heat-treating, material handling and inspection.
- milling
milling
Machining operation in which metal or other material is removed by applying power to a rotating cutter. In vertical milling, the cutting tool is mounted vertically on the spindle. In horizontal milling, the cutting tool is mounted horizontally, either directly on the spindle or on an arbor. Horizontal milling is further broken down into conventional milling, where the cutter rotates opposite the direction of feed, or “up” into the workpiece; and climb milling, where the cutter rotates in the direction of feed, or “down” into the workpiece. Milling operations include plane or surface milling, endmilling, facemilling, angle milling, form milling and profiling.
- part orientation
part orientation
Designing the assembly machine, feeding mechanism and the part itself so the parts to be assembled are properly aligned prior to and during the assembly operation.
- sawing machine ( saw)
sawing machine ( saw)
Machine designed to use a serrated-tooth blade to cut metal or other material. Comes in a wide variety of styles but takes one of four basic forms: hacksaw (a simple, rugged machine that uses a reciprocating motion to part metal or other material); cold or circular saw (powers a circular blade that cuts structural materials); bandsaw (runs an endless band; the two basic types are cutoff and contour band machines, which cut intricate contours and shapes); and abrasive cutoff saw (similar in appearance to the cold saw, but uses an abrasive disc that rotates at high speeds rather than a blade with serrated teeth).
- sintering
sintering
Bonding of adjacent surfaces in a mass of particles by molecular or atomic attraction on heating at high temperatures below the melting temperature of any constituent in the material. Sintering strengthens and increases the density of a powder mass and recrystallizes powder metals.
- web
web
On a rotating tool, the portion of the tool body that joins the lands. Web is thicker at the shank end, relative to the point end, providing maximum torsional strength.