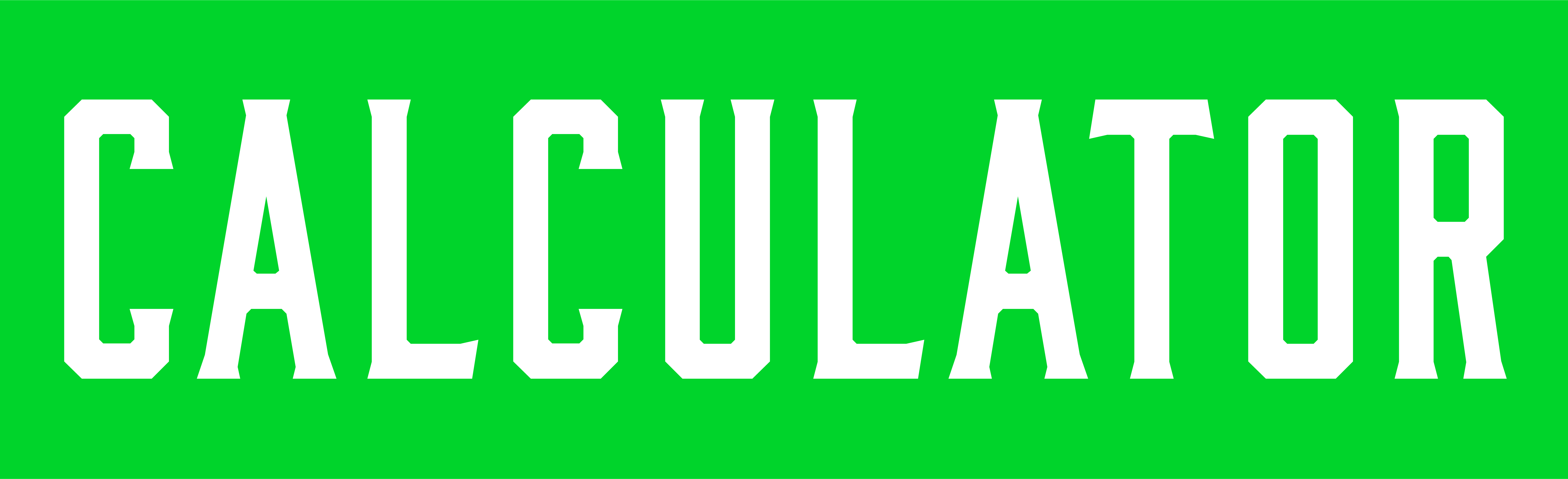
Most materials expand when their temperature increases and contract when their temperature decreases. The size change is approximately proportional to the temperature change, but the amount of size change varies from material to material.
The amount of size change is calculated by the equation:
ΔL = αL0ΔT
where ΔL is the change in length measured along one dimension, α is the coefficient of linear thermal expansion, L0 is the length of the object at the original temperature and ΔT is the temperature change. Both ΔL and L0 have units of length, such as meters, and ΔT has units of degrees, such as ° C, so α has units of 1 ÷ degrees. Alternatively, the units of α could be expressed as m ÷ (m - ° C), for example.
Thermal Expansion Calculator
Formula: ΔL = α × L0 × ΔT
where:
- ΔL is the change in length.
- α is the coefficient of linear thermal expansion.
- L0 is the original length.
- ΔT is the change in temperature.
The coefficient of linear thermal expansion has been measured experimentally for numerous materials. For instance, it is approximately 22.2 × 10-6 ÷ ° C or 12.3 × 10-6 ÷ ° F for aluminum. These two numbers are different because the Fahrenheit and Celsius temperature scales have degrees of different sizes. On the Celsius scale, water freezes at 0° and boils at 100°, so there are 100° between freezing and boiling. On the Fahrenheit scale, water freezes at 32° and boils at 212°, so there are 180° between freezing and boiling. Therefore, 1° F change is smaller than a 1° C change in the ratio of 5:9, which is the ratio of the two coefficients of linear thermal expansion previously noted.
If an aluminum workpiece is 5 ' long at 60° F, and the workpiece temperature changes to 90° F, the length of the workpiece will increase by
ΔL = (12.3 × 10-6 ÷ ° F) × 5 ' × 30° F = 0.00185 ' = 0.022 "
Given that the length changes with the temperature, what length is specified on a drawing? By convention, the length on the drawing is the length when the part is at a uniform temperature of 20° C, or 68° F. If the dimension is measured at another temperature, the length must be corrected using the previous formula before the part is compared to the dimension on the drawing. For that reason, many machine shops find it easier just to measure all dimensions at 20° C. Metrology laboratories are held at 20° C, and shops let parts “soak,” or sit, in the lab until the temperature of the part is 20° C before taking measurements.
Many machine shops claim to hold tolerances significantly tighter than 0.022 " on an aluminum part, even when the temperature in the shop varies more than the example’s 30° F. In a shop, the temperature often varies significantly more on a daily or seasonal basis—a large source of part errors. The situation is generally even more complicated because the machine tool, fixtures, positioning screws and even linear scales change by different amounts with the temperature. The table to which a part is clamped is often made of steel (α = 7.3 × 10-6 ÷ ° F) or cast iron (α = 6.0 × 10-6 ÷ ° F). Some machines use linear scales made of special glass ceramics, which have a low coefficient of linear thermal expansion (on the order of α = 0.06 × 10-6 ÷ ° F) to improve accuracy, but such scales must be carefully mounted to the machine to allow for the difference in the coefficients of linear thermal tool expansion.
In addition, the heat sources in a shop, such as outside air, outside light, shop lighting, operating machines and shop workers, are rarely even or constant.
Recognizing the effects of temperature and coefficient of linear thermal expansion, there are some practical steps to improve accuracy and reduce scrap. The most obvious is air conditioning the shop. While the cost of air conditioning is explicit, the cost of not air conditioning is spread over all of the dimensional tolerance problems a shop encounters. Many shops try to hold “shopping mall-like” conditions, ±3° F.
Windows in a shop are not desirable, because heat from the sunlight can significantly increase the temperature of the shop or machine. Doors should be kept closed whenever possible. Some shops incorporate a temperature lock, using two roll-up doors with a space between them. When material is brought into the shop, it enters through the outer door into the space between the doors. The outer door is then closed, and the material soaks to the temperature of the shop before the inner door is opened.
A large flow of coolant can help keep the machine and workpiece at a more constant temperature. A large coolant tank is beneficial because a large volume of coolant has more capacity to absorb heat without much temperature change. Many shops even control coolant temperature, which is surprisingly easy, inexpensive and effective with a metalworking fluid chiller. CTE
About the Author: Dr. Scott Smith is a professor and chair of the Department of Mechanical Engineering at the William States Lee College of Engineering, University of North Carolina at Charlotte, specializing in machine tool structural dynamics. Contact him via e-mail at kssmith@uncc.edu.
Related Glossary Terms
- ceramics
ceramics
Cutting tool materials based on aluminum oxide and silicon nitride. Ceramic tools can withstand higher cutting speeds than cemented carbide tools when machining hardened steels, cast irons and high-temperature alloys.
- coolant
coolant
Fluid that reduces temperature buildup at the tool/workpiece interface during machining. Normally takes the form of a liquid such as soluble or chemical mixtures (semisynthetic, synthetic) but can be pressurized air or other gas. Because of water’s ability to absorb great quantities of heat, it is widely used as a coolant and vehicle for various cutting compounds, with the water-to-compound ratio varying with the machining task. See cutting fluid; semisynthetic cutting fluid; soluble-oil cutting fluid; synthetic cutting fluid.
- metalworking
metalworking
Any manufacturing process in which metal is processed or machined such that the workpiece is given a new shape. Broadly defined, the term includes processes such as design and layout, heat-treating, material handling and inspection.
- metrology
metrology
Science of measurement; the principles on which precision machining, quality control and inspection are based. See precision machining, measurement.
- tolerance
tolerance
Minimum and maximum amount a workpiece dimension is allowed to vary from a set standard and still be acceptable.