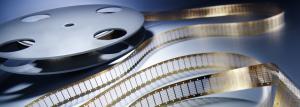
As a precision sheet metal machining technology, photo-chemical etching (PCE) achieves exacting tolerances, is highly repeatable, and in many instances is the only technology that can cost-effectively manufacture precision metal components with the accuracy necessary in demanding and often safety-critical applications.
After design engineers select PCE as their preferred metalworking process, it is important that they fully appreciate not just its versatility, but also the specific aspects of the technology that can affect — and in many instances enhance — product design. This paper analyses what design engineers must appreciate to get the most from PCE, and benchmark the process against other metal machining technologies.
PCE has many attributes that can stimulate innovation and ’stretch the boundaries with the inclusion of challenging product features, enhancements, complexity, and efficiency. It is vital that design engineers fully exploit its potential, and micrometal (incorporating HP Etch and Etchform) advocates that its customers view it as a product development partner — not just a sub-contract manufacturer — early in the design stage allowing OEMs to optimize the potentials that this versatile metal machining process can offer.
General technology attributes
Metal and sheet sizes: Photo etching can be applied to a spectrum of metals in a variety of thicknesses, grades, tempers and sheet sizes. Each supplier can process different thicknesses of metal with different tolerances, and when selecting a PCE partner to work with, it is important to interrogate precisely what capabilities they have.
For example, when working with micrometal’s Etching Group the process can be applied to thin metal sheets ranging from 10 microns to 2000 microns (0.010 mm to 2.00 mm) with a maximum sheet/component size of up to 600 mm x 800 mm. Metals that can be processed include steel and stainless steels, nickel and nickel alloys, copper and copper alloys, tin, silver, gold, Molybdenum, aluminum. and hard to machine metals including highly corrosive materials such as titanium and its alloys.
Standard etching tolerances: Tolerances are a key consideration in any design and with PCE tolerances can vary depending on the material thickness, the material, and the skill and experience of the PCE supplier.
The micrometal Etching Group’s process can produce intricate parts with tolerances as low as ±7 microns depending upon material and its thickness, this tolerance attainment being unique among all alternative metal fabrication technologies. Uniquely, the company uses a special liquid resist system to obtain ultra-thin (2-8 micron) photoresist layers enabling a higher degree of precision in the chemical etching process. It allows the Etching Group to achieve extremely small feature sizes of 25 microns, minimum hole diameter 80 percent of the material thickness, and single-digit micron tolerances repeatably.
As a guide, micrometal’s Etching Group can process stainless steel, nickel, and copper alloys up to 400 micron thick with feature sizes down to 80 percent of material thickness and tolerances of ±10 percent of thickness. Stainless, nickel, and copper above 400-micron thickness and other materials such as tin, aluminum, silver, gold, molybdenum and titanium can have feature sizes down to 120% of material thickness with tolerances of ±10 percent of thickness.
Traditional PCE uses relatively thick dry film resist which compromises ultimate part precision and the available tolerances, and it is only able to achieve 100-micron feature sizes and a minimum hole diameter of 100 to 200 percent material thickness.
In some instances, traditional metal machining technologies can achieve tighter tolerances, but there are limitations. For example, laser cutting can achieve accuracy to 5 percent of the metal thickness, but it is limited to a minimum feature size of 0.2 mm. PCE can achieve a minimum standard feature size of 0.1 mm and openings below 0.050 mm are possible.
In addition, it must be appreciated that laser cutting is a “single point” metal processing technology, which means that it is usually more expensive for complex parts such as meshes, and it cannot achieve the depth/engraved features necessary for fluidic devices like fuel cells and heat exchangers that are easily attained using depth etching.
Burr- and stress-free machining. When it comes to the ability to replicate the exacting accuracy and minimum feature size capabilities of PCE, stamping probably gets the closest, but the trade-off is the stress that is imparted in the metal when it is processed and the residual burrs that are a characteristic of press-working.
Stamped parts need costly post-processing, and as expensive steel tooling is used to produce the parts, it is not viable for short runs. In addition, when processing hard metals, tool wear is an issue, with expensive and time-consuming refurbishment often necessary. Many flexure spring designers and designers of intricate metal components specify PCE due to its burr- and stress-free nature, zero tool wear and speed of supply.
Unique features at no additional cost: Unique characteristics can be designed into products manufactured using photo etching due to the inherent edge “cusp” during the process. By controlling etch cusp a range of profiles can be introduced allowing the manufacture of sharp cutting edges, such as those used in medical blades, or conical openings, such as those used to direct fluid flow in filtration meshes.
Low-cost tooling and design iterations: For OEMs across all industry sectors looking for feature-rich, complex and precise metal parts and components, PCE is now the technology of choice as it not only copes well with difficult geometries, it also allows design engineers flexibility, facilitating the adjustment of designs right up to the point of manufacture.
One major factor that allows for this is the use of digital or glass tooling, which is inexpensive to produce, and therefore inexpensive to change even up to a few minutes before manufacturing commences. Unlike stamping, the cost of digital tooling does not increase with part complexity, which stimulates innovation, as designers focus on optimized part functionality rather than cost.
For traditional metal machining technologies, it is fair to say that increased part complexity equals increased cost, much of this being a product of the costly and intricate tooling. Costs also escalate when traditional technologies have to cope with non-standard materials, thicknesses, and grades, none of which have any effect on the cost of PCE.
As PCE does not use hard tools, distortion and stress are eliminated. Also, components produced are flat, with a clean surface and no burrs as the metal are dissolved away uniformly and evenly until the desired geometries are achieved.
The micrometal company has devised a simple to use the table that helps design engineers review the sampling options available for prototypes of near-series products that can be accessed here.
Economical prototyping: With PCE, users pay by the sheet, not by the part, which means components with different geometries can be processed at the same time from a single tool. The ability to produce many part types in one production run is the key to the enormous cost savings inherent in the process.
PCE can be applied to virtually any metal type whether soft, hard or brittle. Aluminum is renowned for being difficult to stamp due to its softness, and difficult to laser cut due to its reflectivity. Equally, titanium’s hardness can prove challenging. For example, micrometal has developed propriety processes and etching chemistries for both of these specialist materials and is one of the few etching companies in the world with a titanium etching facility.
Add into the mix the fact that PCE is inherently speedy, and the rationale behind the exponential growth in uptake of this technology in recent years is obvious.
Summary
Design engineers are increasingly turning to PCE as they are under pressure to create smaller and increasingly complex precision metal components.
As with any process selection, designers need to be aware of the specific attributes of the chosen manufacturing technology when looking at design attributes and parameters.
The versatility of photo etching and its unique advantages as a precision sheet-metal machining technology makes it an engine for design innovation, and can truly be used to manufacture parts that would be deemed impossible if using alternative metalworking technologies
Related Glossary Terms
- alloys
alloys
Substances having metallic properties and being composed of two or more chemical elements of which at least one is a metal.
- copper alloys
copper alloys
Copper containing specified quantities of alloying elements added to obtain the necessary mechanical and physical properties. The most common copper alloys are divided into six groups, and each group contains one of the following major alloying elements: brasses—major alloying element is zinc; phosphor bronzes—major alloying element is tin; aluminum bronzes—major alloying element is aluminum; silicon bronzes—major alloying element is silicon; copper-nickels and nickel-silvers—major alloying element is nickel; and dilute-copper or high-copper alloys, which contain small amounts of various elements such as beryllium, cadmium, chromium or iron.
- flat ( screw flat)
flat ( screw flat)
Flat surface machined into the shank of a cutting tool for enhanced holding of the tool.
- hardness
hardness
Hardness is a measure of the resistance of a material to surface indentation or abrasion. There is no absolute scale for hardness. In order to express hardness quantitatively, each type of test has its own scale, which defines hardness. Indentation hardness obtained through static methods is measured by Brinell, Rockwell, Vickers and Knoop tests. Hardness without indentation is measured by a dynamic method, known as the Scleroscope test.
- metalworking
metalworking
Any manufacturing process in which metal is processed or machined such that the workpiece is given a new shape. Broadly defined, the term includes processes such as design and layout, heat-treating, material handling and inspection.
- micron
micron
Measure of length that is equal to one-millionth of a meter.
- stainless steels
stainless steels
Stainless steels possess high strength, heat resistance, excellent workability and erosion resistance. Four general classes have been developed to cover a range of mechanical and physical properties for particular applications. The four classes are: the austenitic types of the chromium-nickel-manganese 200 series and the chromium-nickel 300 series; the martensitic types of the chromium, hardenable 400 series; the chromium, nonhardenable 400-series ferritic types; and the precipitation-hardening type of chromium-nickel alloys with additional elements that are hardenable by solution treating and aging.
- titanium nitride ( TiN)
titanium nitride ( TiN)
Added to titanium-carbide tooling to permit machining of hard metals at high speeds. Also used as a tool coating. See coated tools.
- tolerance
tolerance
Minimum and maximum amount a workpiece dimension is allowed to vary from a set standard and still be acceptable.
- turning
turning
Workpiece is held in a chuck, mounted on a face plate or secured between centers and rotated while a cutting tool, normally a single-point tool, is fed into it along its periphery or across its end or face. Takes the form of straight turning (cutting along the periphery of the workpiece); taper turning (creating a taper); step turning (turning different-size diameters on the same work); chamfering (beveling an edge or shoulder); facing (cutting on an end); turning threads (usually external but can be internal); roughing (high-volume metal removal); and finishing (final light cuts). Performed on lathes, turning centers, chucking machines, automatic screw machines and similar machines.