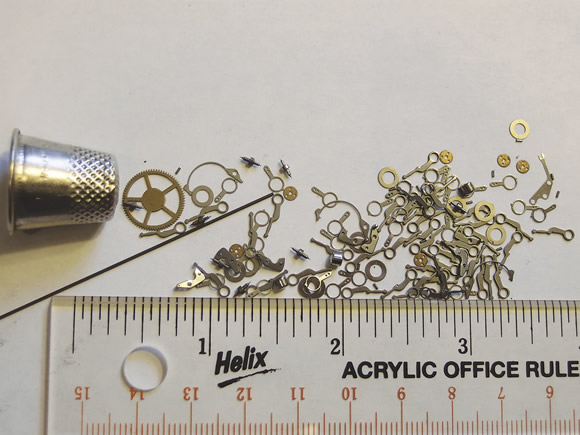
Courtesy of L. Gillespie
These microparts from Glen Mills, including micropins in the upper right-hand corner and stainless steel pivot gear blanks near the thimble, were finished with a Turbula shaker/mixer.
Four deburring methods for mass finishing microparts.
Deburring parts smaller than ⅛" via mass finishing poses several challenges. Part manufacturers that are used to working exclusively with larger parts need to confront these challenges when deburring microparts.
In addition to the difficulties posed by part size, lightweight surface tension and electrostatic charges can retard the motion of microparts and deburring media. The biggest impediment to effective deburring, though, is tumbling media that’s too large.
Three rules should be followed when mass finishing microparts: Keep the burr thin, use a process and media targeted for microscale parts and optimize the separation of parts and media.
Consider Figure 1. The data is typical of all mass-finishing operations, although finishing time and results will vary by application. In this example, it takes 30 minutes to remove a 0.003"-thick burr from a stainless steel part. As the burr is removed, a radius forms on the part’s edge and grows to about 0.003" (76.2µm) after 30 minutes. The surface roughness improves during finishing, with the outer part surfaces being reduced by 0.0001" (2.54µm).
Burr thickness determines removal time, and that time determines the final edge radius. Making thinner burrs shortens cycle times and lessens the impact on part features. If burr thickness can be kept to 0.000050" (1.27µm) or thinner, a light tumbling action and short cycle will remove it.
Because the part features are microsized, it is essential to use tumbling media that are smaller than the part. As a rule of thumb, use tumbling media that are at least one-fourth the size of the feature to be deburred. However, when the media are very small, time becomes a major consideration.
Studies have been conducted on ½"-dia., 1018 steel cylinders that were centrifugally tumbled in ¼", plastic, pyramid-shaped media. The cylinders developed a radius according to the formula R=0.00281t0.33, where t is time in minutes, while 0.115" (2.92mm) cylinders tumbled in No. 14 aluminum oxide (0.053", or 1.35mm, particles) had a radius defined by R=0.00076t0.41. That is a factor of 3.7 slower radii generation when using smaller media on a smaller part. When even smaller parts and smaller media are involved, the time requirements can be 10 to 100 times longer than for ¼ " or larger parts.
The reason for slow cutting when using small media on microparts can be envisioned with the numbers in Table 1 (page 83). As seen in the table, a 0.001"-dia. pin weighs 1/61,600th as much as a ¼"-dia. pin. So, the force between a deburring particle and the part is far less than for a ¼" pin. The cutting ability is actually much different than that because in a conventional tumbling operation, the fine grains of deburring media support each other, and the part tumbles as if in a glob of mashed potatoes.
Presuming the burrs are kept to a thickness of about 0.001" (25.4μm) or less, there are four reasonably good choices for removing burrs: centrifugal barrel deburring, conventional vibratory deburring in palm-sized containers, media mixers and shakers, and magnetic-abrasive finishing.
Centrifugal Deburring
In centrifugal barrel finishing, a small barrel containing the parts and tumbling media rotates, causing the particles to rub against the part edges and remove burrs. The gravitational force between parts and media when centrifugal deburring is 10 to 25 times greater than when vibratory finishing, because the barrels are rotating on a long arm that is also rotating, which provides additional centrifugal grinding force. More force equals faster deburring.
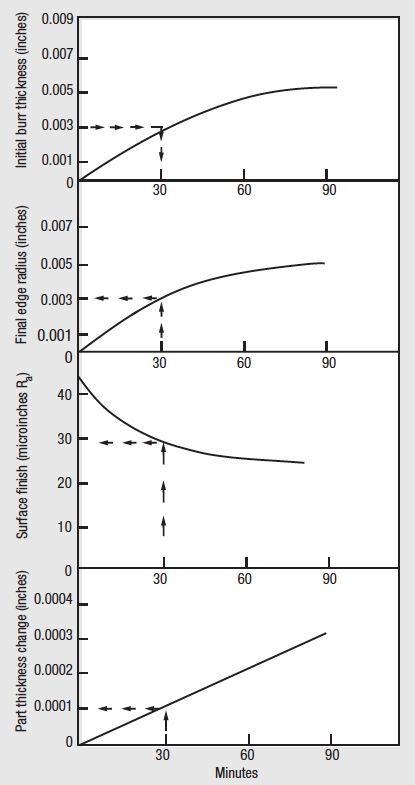
Courtesy of L. Gillespie
Figure 1. Four changes in part finish imparted by mass-finishing processes. Arrows indicate properties that can be achieved in 30 minutes. For example, a 0.003" burr can be removed in 30 minutes (top chart). During 30 minutes of deburring, the other three characteristics will also change as indicated by the arrows.
The key is to find centrifugal machines that use small barrels, so that separating parts from media can be accomplished more quickly. For microparts, the barrels typically should be pint- or cup-sized.
Figure 2 illustrates a small, 303 stainless steel pin that was centrifugal-barrel-finished for 20 minutes with No. 14 fused Al2O3 as the media at a finishing force of 15 G. The initial height and thickness of the burrs are shown. After centrifugal finishing, the part was burr-free at 10× magnification and the radius produced was less than 0.005" (127μm). The cut-off burr is not removed in deburring. Most microparts require 15 to 20 G, and, because the microparts will be smaller than the part used in Figure 2, will need No. 24 or smaller media.
Smaller media will require longer deburring times if the burrs are the same size as shown in Figure 2. A No. 24 particle has an average size of 0.0270" (0.69mm); a No. 80 has an average size of 0.0065" (165μm).
Most centrifugal machines are designed for larger parts and much higher volumes. But at least two companies—Bel-Air in North Kingston, R.I., and DB Importers of Blanchester, Ohio, which distributes Tipton machines—provide barrel sizes of about a liter, which is appropriate for most microscale work. The equipment is simple to run and most machines will last for decades.
Vibratory Finishing
Vibratory finishers vibrate a container of parts and media in such a manner that the mixture rolls. This allows the media to rub over the parts and remove burrs—much like waves and sand that slowly smooth the sharp edges of driftwood and stones on a beach.
Table 1
Part diameter (in.) |
Part volume/length (in.3/in.) |
Weight (lb./in.) |
Weight (gm/in.) |
0.001 |
0.008 × 10-4 |
0.0025 × 10-4 |
0.0001 |
0.020 |
3.41 × 10-4 |
0.9889 × 10-4 |
0.0449 |
0.125 |
133.4 × 10-4 |
38.696 × 10-4 |
1.7563 |
0.250 |
533.6 ×10-4 |
154 × 10-4 |
7.0252 |
Pint-sized vibratory finishers are commercially available for about $150. They facilitate the development of prototype deburring processes, allowing the user to validate that part geometry is correct and burrs can be removed without adversely affecting other features.
Pint-size vibratory finishers may not provide as fast a production cycle as needed, but they can prove whether or not mass finishing will work for the parts in question.
One high-production machine from Polyservice AG, Lengnau, Switzerland, incorporates 10 containers, some as small as 0.38 pints (0.21 liters). This lets the user deburr 10 batches of parts at a time or test different media.
A small vibratory tumbler from Raytech Metal Finishing, Middletown, Conn., might also be a good choice for microparts.
Mixers and Shakers
The Turbula machine, which has been used by Swiss watchmakers since 1950 for deburring delicate, small parts, is a vibratory-powder or -liquid mixer featuring a unique 3-D, figure-8 mixing action. Available from Glen Mills Inc., Clifton, N.J., the machine combines and moves abrasives, surfactants, grinding media and other deburring compounds to generate a homogeneous action on all part surfaces. In 15 hours of unattended motion, a 1-liter container on the Turbula can deburr 5,000 to 10,000 delicate watch parts. (It can also deburr other microparts.)
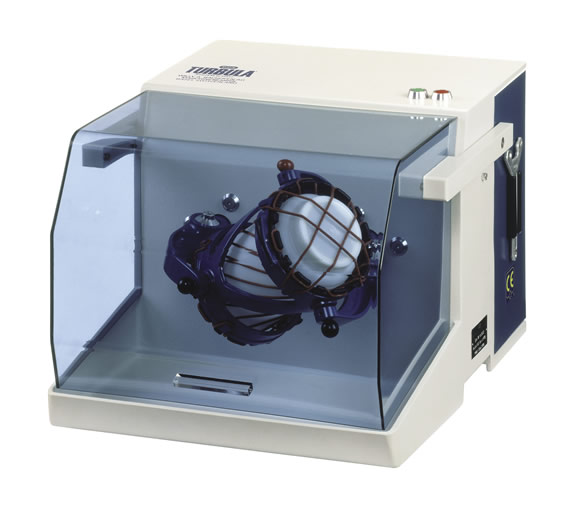
Courtesy of Glen Mills
The Turbula Model T2F shaker/mixer tabletop machine is available from Glen Mills.
Shakers and mixers are suited to the needs of micromanufacturing because they provide predictable edge quality. Available containers range from thimble- to pint-sized.
Magnetic Abrasives
The last approach is to apply magnetic-abrasive particles. In one case, this technique removed a 0.008" (0.2mm) tall burr in about 1 minute, although a more typical cycle time is 5 minutes.
Equipment for this process is made by Dr. Hitomi Yamaguchi Greenslet at the University of Florida. With this technique, two variations of equipment can be used. In the first, a magnetic part is moved through abrasive particles by an oscillating or rotating magnetic field.
The second variation is used when the parts are nonmagnetic. In these cases, an abrasive-coated magnetic material, or a simple mixture of magnetic media and conventional abrasive slurry, can be magnetically moved past the parts. The smallest media size used in this process is typically about 0.002" (50μm). Most of the applications involve holding the part in some manner and moving the media, but the original concept was more like conventional mass-finishing operations.
In a slightly different application, features measuring 0.0002" to 0.0008" (5µm to 20μm) on a MEMS device were polished. The part was submersed in a homogeneous fluid mixture, called a “magnetic abrasive fluid mixture,” consisting of abrasive particles and ferrous particles. An alternating magnetic field actuated the MAFM into an oscillatory motion. The reciprocating MAFM flowed through the micropores or past the microfeatures and polished the part.
The MAFM process is one step beyond the more-traditional magnetic-abrasive finishing processes mentioned earlier, and it provides the next level in precision for the micro world. But it requires part fixturing, whereas conventional magnetic-abrasive finishing allows parts to either be fixtured or handled in mass.
Because magnetic force fields are limited by distance, containers smaller than 1 pint are advantageous and a good choice for microparts.
Off-the-shelf, commercial-grade magnetic-abrasive equipment is available on a limited basis. The cost of custom equipment available from Greenslet is equal to or below the cost of commercial centrifugal machines.
Most sellers of deburring equipment will run tests on customers’ and prospects’ parts. Those interested in conducting their own in-house tests, however, have a number of options. The least-expensive way is to invest about $175 in a 0.05-cu.-ft., tabletop vibratory machine.
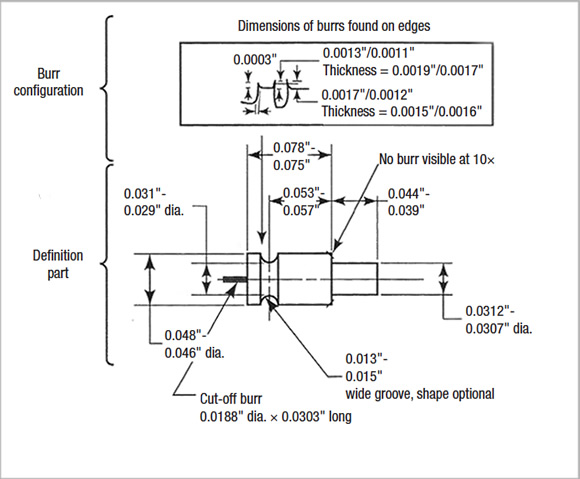
Courtesy of Industrial Press. Original data by L. Gillespie.
Figure 2. Schematic of a stainless steel micropart that is to be centrifugal-barrel-finished.
The next option is the centrifugal method. It will do the same work as the vibratory unit, but in a tenth to a hundreth of the time. Centrifugal systems are the preferred route for most companies. The tradeoff is the equipment’s $10,000 to $25,000 price tag.
Vibratory and centrifugal machines are economical and simple to use. The newer technologies, such as magnetic-abrasive deburring and polishing, are still being developed for micro parts and features. The equipment is clearly designed for specific geometries, and it is one of the few processes truly tuned to micro parts and features.
Magnetic-abrasive machines cost between $10,000 and $25,000, but they can be challenging to acquire and operate. Not many commercial models are available in the U.S., and media must either be imported from Japan or be custom-made in the U.S.
Once you’ve decided on the type of deburring machine to operate, another consideration when bringing the process in-house is finding an effective solution for separating the parts from the media. That can be done with vibratory bowl feeders for many parts, but microparts may require using specially designed feeders/separators or working with the supplier to tune the vibratory bowl parts feeder to the weight, volume and geometry of the parts you are putting into them. Electrostatic, magnetic or surface tension may also be used to separate parts from media. CTE
About the Author: Dr. LaRoux K. Gillespie has a 40-year history with precision part production as an engineer and manager. He is the author of 12 books on deburring and more than 220 technical reports and articles on precision machining. E-mail: laroux1@earthlink.com.
Contributors
Glen Mills Inc.
(973) 777-0777
www.glenmills.com
Polyservice AG
+41-32-653-04-44
www.polyservice.ch
Raytech Metal Finishing
(860) 632-2020
www.raytechmetalfinishing.com
University of Florida
(352) 392-3261
www.ufl.ed
Related Glossary Terms
- 3-D
3-D
Way of displaying real-world objects in a natural way by showing depth, height and width. This system uses the X, Y and Z axes.
- abrasive
abrasive
Substance used for grinding, honing, lapping, superfinishing and polishing. Examples include garnet, emery, corundum, silicon carbide, cubic boron nitride and diamond in various grit sizes.
- aluminum oxide
aluminum oxide
Aluminum oxide, also known as corundum, is used in grinding wheels. The chemical formula is Al2O3. Aluminum oxide is the base for ceramics, which are used in cutting tools for high-speed machining with light chip removal. Aluminum oxide is widely used as coating material applied to carbide substrates by chemical vapor deposition. Coated carbide inserts with Al2O3 layers withstand high cutting speeds, as well as abrasive and crater wear.
- barrel finishing
barrel finishing
Mass finishing process that involves low-pressure abrasion resulting from tumbling workpieces in a barrel (usually of hexagonal or octagonal cross section) together with an abrasive slurry. See finishing.
- burr
burr
Stringy portions of material formed on workpiece edges during machining. Often sharp. Can be removed with hand files, abrasive wheels or belts, wire wheels, abrasive-fiber brushes, waterjet equipment or other methods.
- grinding
grinding
Machining operation in which material is removed from the workpiece by a powered abrasive wheel, stone, belt, paste, sheet, compound, slurry, etc. Takes various forms: surface grinding (creates flat and/or squared surfaces); cylindrical grinding (for external cylindrical and tapered shapes, fillets, undercuts, etc.); centerless grinding; chamfering; thread and form grinding; tool and cutter grinding; offhand grinding; lapping and polishing (grinding with extremely fine grits to create ultrasmooth surfaces); honing; and disc grinding.
- parts feeder
parts feeder
Mechanism that delivers parts to the assembly machine at a specified rate and with proper orientation.
- polishing
polishing
Abrasive process that improves surface finish and blends contours. Abrasive particles attached to a flexible backing abrade the workpiece.
- precision machining ( precision measurement)
precision machining ( precision measurement)
Machining and measuring to exacting standards. Four basic considerations are: dimensions, or geometrical characteristics such as lengths, angles and diameters of which the sizes are numerically specified; limits, or the maximum and minimum sizes permissible for a specified dimension; tolerances, or the total permissible variations in size; and allowances, or the prescribed differences in dimensions between mating parts.