Contact Details
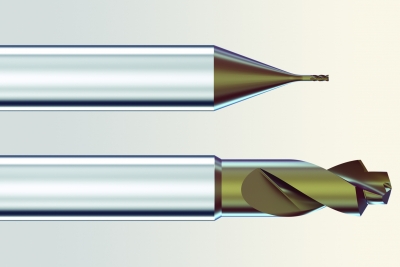
A drill, a milling cutter, an ultimate machining strategy with perfectly tuned parameters; this is needed, when machining medical screws made from titanium or stainless steel. Mikron Tool developed a turnkey solution, which simultaneously does the machining of hex lobular bone screws (better known as Torx) 50% faster, guaranteeing also a high degree of precision of the profile and achieving nearly burr-free results.
With an increasing tendency, yearly 900 million medical screws are produced. Each second which can be gained in the process counts. Most of these screws are produced on lathe machines, on the main spindle the thread and on the subspindle the hexalobular Torx socket is machined. That particular operation is usually slower and defines the cycle time. Here is an opportunity to improve the efficiency, all be it by only a few seconds. Nevertheless it saves the manufacturer time and money.
Mikron Tool offers with the Hexalobe-Program a turnkey solution, which is not only based on the cutting tools, but also delivers a package with the best machining strategy and the right parameters. Because during the development of the program it became quite clear that good results can only be obtained when all factors fall in place correctly.
The two materials with which today 90% of all medical screws are made are titanium and stainless steel. These are quite different when it comes to their behavior during the chip-removal process. Such that during the development it became clear that the tools as well as the suitable strategy needed a differentiation. Especially with titanium with its high elasticity stronger cutting force was required, which in turn produced higher stress on the cutting edges. This already meant right from the beginning of the development, that a different carbide was called for.
For the machining process, both materials have to be differentiated. While with titanium the helical interpolation and also side milling are possible, Mikron Tool only recommends side milling when working with stainless steel. Even though this particular process is a bit more time consuming.
A well-defined strategy was just as important as the cutting tools when development was initiated. Because when choosing the tools it must be clear how these are being applied.
Right from the beginning different approaches were considered. Especially efficient turned out to be the one that could do with a minimum of different tools, i.e. Step drilling – milling the hexalobe – subsequent deburring.
Other strategies like predrilling of the six exterior diameters and the center with the following milling and deburring were a challenge, especially when working with titanium. It turned out to be a demanding task for the microdrills.
The milling process, in the chosen strategy the most time consuming operation, can be done with helical interpolation (with max. pitch 0.8 x d) or with side milling (with stepover max. 0.5 x d).
Each procedure has its pros and cons: With helical interpolation there will be a slight lateral load at the milling cutter (Fx), since there is also a simultaneous vertical stress (Fz).
A higher stress on the cutting edge corners has to be reckoned with and because of this they were geometrically reinforced. Generally this produces a faster and more fluid operation. With the side milling (or wall milling) the total depth is reached in several steps, since only two axes are used for each step. The step-over stays the same for the entire operation even though the radial stress on the cutter is more elevated.
The four operations (pre-hole drilling – chamfering – milling – deburring) need to be completed in three operations with two tools. First a step drill does the center bore and a chamfer of 120 degrees and this right away with an excellent surface quality of Ra 0.2 um, Rz 0.8 um. After the milling of the final shape, this tool will be used once again in the last operation to achieve a nearly burr-free surface and superior surface quality. The milling cutter with 3 or 4 flutes – depending on diameter – is available in two standard lengths, so that depending of the screw type, different Torx shapes can be machined. It’s high stiffness allows machining with high feeds and massive stepover, while still guaranteeing the necessary hexalobular profile accuracy. The surface quality is given by the special geometry which also affords to work with high stepover so that the operation can be completed with few steps.
Besides efficient machining, which means short cycle time and long tool life, the development of the new tools concentrated also on achieving top quality. Tight tolerances must be kept to guarantee profile accuracy and wall squareness of the hexalobular socket, and this from the first to the last screw produced.
Obviously the surface quality and burr condition are also an important topic.
The head of development, Alberto Gotti, confirms that there is no “perfect solution for all cases.”
Even though, this turnkey program with a combination of cutting tools, recommendations for the strategy and parameters gives the end-user excellent support, he still challenged to find “his” balance between most efficient machining, high precision and quality.
First experiences with the new CrazyDrill and CrazyMill Hexalobe tools on a lathe machine 32/8 from DMG Mori have shown, that cycle time compared to other on the market available tools could be reduced by 50% to 60% while at the same time with a fast feed (0.03mm/rev. per flute) and high surface quality (Ra = 0.2 um) can be reached.
Related Glossary Terms
- burr
burr
Stringy portions of material formed on workpiece edges during machining. Often sharp. Can be removed with hand files, abrasive wheels or belts, wire wheels, abrasive-fiber brushes, waterjet equipment or other methods.
- chamfering
chamfering
Machining a bevel on a workpiece or tool; improves a tool’s entrance into the cut.
- cutting force
cutting force
Engagement of a tool’s cutting edge with a workpiece generates a cutting force. Such a cutting force combines tangential, feed and radial forces, which can be measured by a dynamometer. Of the three cutting force components, tangential force is the greatest. Tangential force generates torque and accounts for more than 95 percent of the machining power. See dynamometer.
- feed
feed
Rate of change of position of the tool as a whole, relative to the workpiece while cutting.
- flutes
flutes
Grooves and spaces in the body of a tool that permit chip removal from, and cutting-fluid application to, the point of cut.
- gang cutting ( milling)
gang cutting ( milling)
Machining with several cutters mounted on a single arbor, generally for simultaneous cutting.
- interpolation
interpolation
Process of generating a sufficient number of positioning commands for the servomotors driving the machine tool so the path of the tool closely approximates the ideal path. See CNC, computer numerical control; NC, numerical control.
- lathe
lathe
Turning machine capable of sawing, milling, grinding, gear-cutting, drilling, reaming, boring, threading, facing, chamfering, grooving, knurling, spinning, parting, necking, taper-cutting, and cam- and eccentric-cutting, as well as step- and straight-turning. Comes in a variety of forms, ranging from manual to semiautomatic to fully automatic, with major types being engine lathes, turning and contouring lathes, turret lathes and numerical-control lathes. The engine lathe consists of a headstock and spindle, tailstock, bed, carriage (complete with apron) and cross slides. Features include gear- (speed) and feed-selector levers, toolpost, compound rest, lead screw and reversing lead screw, threading dial and rapid-traverse lever. Special lathe types include through-the-spindle, camshaft and crankshaft, brake drum and rotor, spinning and gun-barrel machines. Toolroom and bench lathes are used for precision work; the former for tool-and-die work and similar tasks, the latter for small workpieces (instruments, watches), normally without a power feed. Models are typically designated according to their “swing,” or the largest-diameter workpiece that can be rotated; bed length, or the distance between centers; and horsepower generated. See turning machine.
- milling
milling
Machining operation in which metal or other material is removed by applying power to a rotating cutter. In vertical milling, the cutting tool is mounted vertically on the spindle. In horizontal milling, the cutting tool is mounted horizontally, either directly on the spindle or on an arbor. Horizontal milling is further broken down into conventional milling, where the cutter rotates opposite the direction of feed, or “up” into the workpiece; and climb milling, where the cutter rotates in the direction of feed, or “down” into the workpiece. Milling operations include plane or surface milling, endmilling, facemilling, angle milling, form milling and profiling.
- milling cutter
milling cutter
Loosely, any milling tool. Horizontal cutters take the form of plain milling cutters, plain spiral-tooth cutters, helical cutters, side-milling cutters, staggered-tooth side-milling cutters, facemilling cutters, angular cutters, double-angle cutters, convex and concave form-milling cutters, straddle-sprocket cutters, spur-gear cutters, corner-rounding cutters and slitting saws. Vertical cutters use shank-mounted cutting tools, including endmills, T-slot cutters, Woodruff keyseat cutters and dovetail cutters; these may also be used on horizontal mills. See milling.
- pitch
pitch
1. On a saw blade, the number of teeth per inch. 2. In threading, the number of threads per inch.
- step-over
step-over
Distance between the passes of the toolpath; the path spacing. The distance the tool will move horizontally when making the next pass. Too great of a step-over will cause difficulty machining because there will be too much pressure on the tool as it is trying to cut with too much of its surface area.
- stiffness
stiffness
1. Ability of a material or part to resist elastic deflection. 2. The rate of stress with respect to strain; the greater the stress required to produce a given strain, the stiffer the material is said to be. See dynamic stiffness; static stiffness.