You may not have heard, but ultrasonic waves can help machine precise features into hard, brittle materials.
Ultrasonic machining (USM) goes back more than 50 years, but there are relatively few practitioners today. Bullen Inc. is an exception. In the USM process used by the Eaton, Ohio-based contract manufacturer, a low-frequency electrical signal is sent to a transducer, which converts the electrical energy into mechanical motion. This high-frequency, low-amplitude vibratory motion is acoustically transmitted to a custom tool, typically made from steel, which experiences linear oscillation. Tool motion is usually less than 0.002" (0.508mm) at a rate of about 20 kHz.
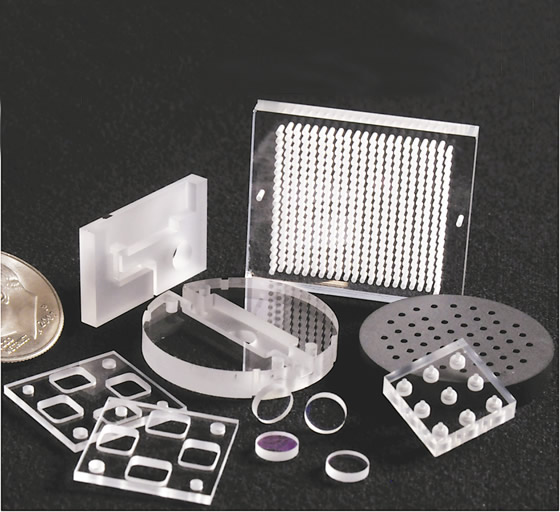
Ultrasonic machining can cut glass, sapphire, engineered ceramics, silicon carbide, quartz, single-crystal materials, PCD, ferrite, graphite, glassy carbon, composites and piezoceramics, among other materials.
While the tool moves, a slurry flows between it and the workpiece. The slurry consists of abrasive grains suspended in water or a chemical solution. Abrasives include diamond, alumina, boron carbide and silicon carbide, with the abrasive chosen dependent on the hardness of the workpiece material. The harder the workpiece, the harder the abrasive, noted Greg Fitch, a business unit manager at Bullen.
That’s because the abrasive grains, not the tool, contact the workpiece during USM. As the tool oscillates, it hammers the grains into the workpiece, abrading it and eventually leaving a precise reverse image of the tool shape.
Low force is applied to the grains, causing microscopic fracturing of the workpiece that removes material. The amount of force applied depends on the tool or application. Small abrasive grains, for example, are accelerated at many thousands of “g” forces. The result, Fitch said, is significantly less subsurface damage compared to conventional machining alternatives, such as EDMing or drilling with a PCD tool. This, in turn, reduces the likelihood of workpiece fractures that might lead to device failure. Also, USM is nonthermal, eliminating the risk of heat-related damage that can occur when laser machining.
Process Advantages
According to Bullen, USM also stacks up well against conventional machining methods when creating features. Machining with PCD tools, for example, limits feature sizes and shapes. EDMing offers more feature options but can’t be used on nonconductive materials.
USM, on the other hand, can machine nonconductive materials. Holes of any shape are the most common USM feature, according to Murali Sundaram, director of the Micro and Nano Manufacturing Laboratory at the University of Cincinnati.
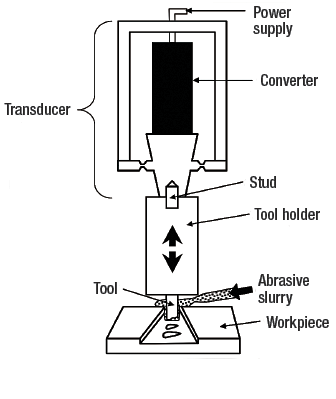
In an ultrasonic machine tool (above), high-frequency, low-amplitude energy is transmitted to the tool assembly. A constant stream of abrasive slurry passes between the tool and workpiece (below). The vibrating tool, combined with the abrasive slurry, uniformly abrades the material, leaving a precise reverse image of the tool shape in the workpiece.
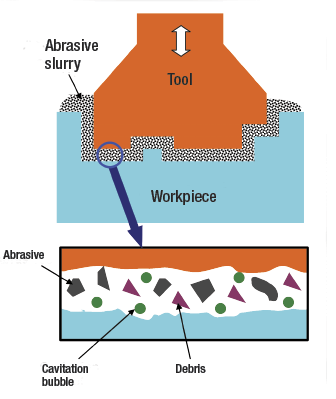
Bullen’s USM also can create cavities of varying depths, as well as OD and ID features. Through-holes and flat-bottomed cavities constitute nearly all of Bullen’s USM work.
The USM process can produce features as small as 0.006 " (0.152mm). Factors that determine feature size include the size of the abrasive grains and tool-tip design, according to Sundaram.
Fitch said Bullen’s USM process can hold tolerances as tight as ±0.001 " (±25µm) and achieve aspect ratios as high as 25:1, under normal circumstances, depending on material type and feature size. In certain cases, the company has been able to push aspect ratios as high as 60:1, Fitch reported.
The key to producing accurate features is the USM tool, according to Thomas Fote, Bullen’s director of business development. These tools can incorporate hundreds—or even thousands—of drill points, allowing USM users to save time by drilling large numbers of features in a single machining step.
Tool Challenge
The main challenge is designing and making a tool so that it delivers the desired results. The company machines its tools in-house using processes that include CNC machining and EDM-ing, Fote said, adding that it also designs and makes all of its own USM equipment.
“If you’ve got 2,000 drill points on a tool, they all need to be doing the right thing,” Fote said. “If 1,900 of them are doing the right thing but 100 aren’t, you’re going to have problems.”
USM is suitable for machining materials harder than 40 HRC, including glass, sapphire, engineered ceramics, silicon carbide, quartz, single-crystal materials, PCD, ferrite, graphite, glassy carbon, composites and piezoceramics. “Generally speaking, we don’t machine metals or plastics,” Fote said.
“Not many people can drill sapphire, silicon carbide or even silicon and create a cost-effective part, whereas we can,” Fitch said, adding that doing so via USM may take 10 percent of the machining time of conventional methods.
Common USM applications include semiconductors, MEMS devices and microfluidic components. When using USM, a number of small parts can be fixtured together in an array and machined simultaneously.
Bullen mainly USMs through-hole arrays in glass constraint wafers for MEMS pressure sensors. Machining large numbers of through-holes simultaneously is significantly less expensive on a per-die basis than conventional step-and-repeat processes, according to the company.
USM Downsides
For some applications, however, USM won’t be the right choice. Those involving small numbers of parts, for example, normally are not a good fit because of the amount of tooling design, Fitch said. Making the necessary tooling for a job can take from a day to a few weeks, but typically takes a week or less.
Another factor to consider when evaluating USM is the number of features to be machined. “We have more competition when there are a smaller number of holes in a substrate,” Fitch said. “If you’re making a part with two holes in it, it may be cheaper to diamond-drill.”
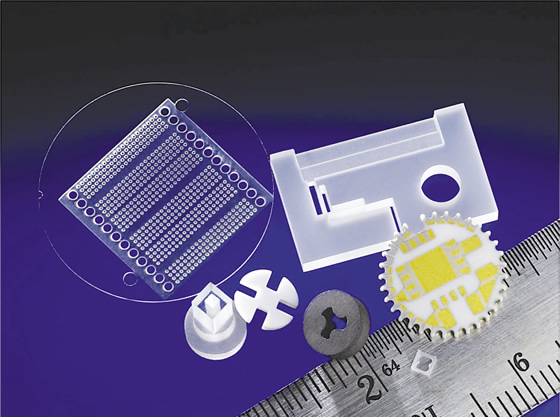
Bullen’s USM process can hold tolerances as tight as ±0.001".
In addition, tolerances produced by USM may not be tight enough for MEMS and some other micromanufacturing applications, Fote said. “There are applications where people are looking for tolerances of a few microns.”
Although few part manufacturers perform ultrasonic machining, Sundaram thinks the process is well-positioned for the future, given the increasing use of advanced ceramics, composites and other modern engineering materials that match up well with its capabilities.
Bullen, for example, reported that it has had some success applying USM to ceramic-metal composites that exhibit some brittleness. “As these advanced materials become more popular,” Fote said, “there will be more demand for ultrasonic machining.” CTE
About the Author: William Leventon is a New Jersey-based freelance writer. He has an M.S. in Engineering from the University of Pennsylvania and a B.S. in engineering from Temple University. Telephone: (609) 926-6447. E-mail: wleventon@verizon.net.
Related Glossary Terms
- abrasive
abrasive
Substance used for grinding, honing, lapping, superfinishing and polishing. Examples include garnet, emery, corundum, silicon carbide, cubic boron nitride and diamond in various grit sizes.
- ceramics
ceramics
Cutting tool materials based on aluminum oxide and silicon nitride. Ceramic tools can withstand higher cutting speeds than cemented carbide tools when machining hardened steels, cast irons and high-temperature alloys.
- composites
composites
Materials composed of different elements, with one element normally embedded in another, held together by a compatible binder.
- computer numerical control ( CNC)
computer numerical control ( CNC)
Microprocessor-based controller dedicated to a machine tool that permits the creation or modification of parts. Programmed numerical control activates the machine’s servos and spindle drives and controls the various machining operations. See DNC, direct numerical control; NC, numerical control.
- ferrite
ferrite
Solid solution of one or more elements in body-centered cubic iron. Unless otherwise designated, for instance, as chromium ferrite, the solute is generally assumed to be carbon. On an equilibrium diagram, there are two ferrite regions separated by an austenite area. The lower area is alpha ferrite and the upper area is delta ferrite. If there is no designation, alpha ferrite is assumed. Not more than 0.04 percent carbon can be dissolved in alpha iron. Ferrite is stable below 1,670º F (910º C); it is soft, highly ductile, and magnetic. Ferrite loses its magnetic property above 1,414º F (768º C).
- hardness
hardness
Hardness is a measure of the resistance of a material to surface indentation or abrasion. There is no absolute scale for hardness. In order to express hardness quantitatively, each type of test has its own scale, which defines hardness. Indentation hardness obtained through static methods is measured by Brinell, Rockwell, Vickers and Knoop tests. Hardness without indentation is measured by a dynamic method, known as the Scleroscope test.
- inner diameter ( ID)
inner diameter ( ID)
Dimension that defines the inside diameter of a cavity or hole. See OD, outer diameter.
- laser machining
laser machining
Intensified, pulsed beams of light generated by lasers—typically carbon dioxide or neodium-doped yttrium aluminum garnet (Nd:YAG)—that drill, weld, engrave, mark, slit and caseharden. Usually under CNC, often at both high cutting rates (100 linear in./sec.) and high power (5kW or more). Lasers also are used in conjunction with in-process quality-control monitoring systems allowing measuring accuracies of 0.00001".
- outer diameter ( OD)
outer diameter ( OD)
Dimension that defines the exterior diameter of a cylindrical or round part. See ID, inner diameter.
- polycrystalline diamond ( PCD)
polycrystalline diamond ( PCD)
Cutting tool material consisting of natural or synthetic diamond crystals bonded together under high pressure at elevated temperatures. PCD is available as a tip brazed to a carbide insert carrier. Used for machining nonferrous alloys and nonmetallic materials at high cutting speeds.
- through-hole
through-hole
Hole or cavity cut in a solid shape that connects with other holes or extends all the way through the workpiece.
- ultrasonic machining
ultrasonic machining
Material-removal operation in which an abrasive slurry flows between a tool, vibrating at a high frequency, and a workpiece.